A Detailed Guide to The Design and Installation of Complete Off-Grid Solar Power for a Home
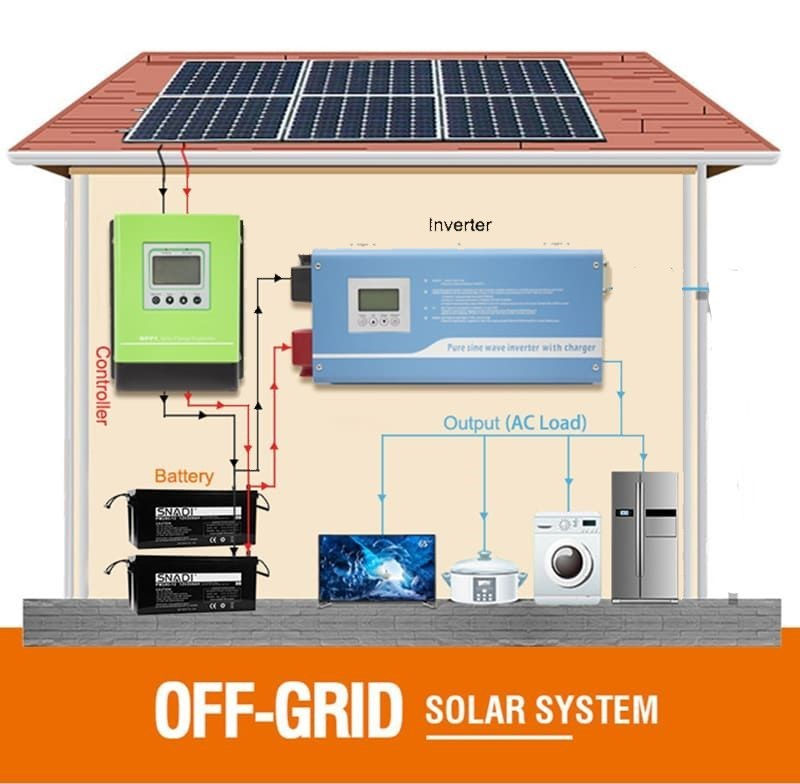
What is meant by off-grid solar systems?
With an off-grid solar system, you’re completely reliant on the sun and energy stored in batteries to power your home or business.
While an on-grid (also known as grid-tied) solar energy system is connected to the utility grid, an off-grid solar energy system is not.
OFF-GRID SOLAR SYSTEM DESIGN & INSTALLATION
Design and installation of solar power (OFF-GRID) for a home to power the following electrical appliances.
22 x Light bulbs, 5 x Electric fans, Air conditioner, 55 inches Television, Sound system, Washing machine, Refrigerator, Freezer, 2 x laptops
Here's a general outline for designing a solar power system to run the appliances listed. We decided to go deeper for those who want to learn about solar power sizing in detail and hope that you will find some of the answers to your questions.
Electrical Load Detail:
2000W Air conditioner use for 7 hours/day
110W Television use for 5 hours/day
170W Refrigerator use for 24 hours/day (because of cycle on and off throughout the day say 18 hours/day)
275W Freezer use for 24 hours/day (because of cycle on and off throughout the day say 18 hours/day)
2 x 95W Laptop use for 4 hours/day
5 x 75W Electric fan use for 10 hours/day
200W Sound system use for 1 hour/day
20 x 10W Light bulbs use for 8 hours/day
2400W Washing machine use for 1 hour/day
Solar System Detail:
Solar System Voltage (As per Battery Bank) = 48V DC
Loose Wiring Connection Factor = 20%
Daily Peak Sunshine Hour = 6 Hours/Day
Inverter Detail:
Future Load Expansion Factor = 25%
Inverter Efficiency = 80%
Inverter Power Factor = 0.8
Safety Factor = 1.25
Calculation:
Step-1: Calculate Electrical Usages per Day (Energy usage per Day)
To know the amount of energy (kWh) consumed each day by a particular appliance.
We simply multiply the total wattage (W) of the appliance by the number of hours (T) you use it.
kWh = n x W x T
where n = number of each particular appliance
Power Consumption for Air conditioner = No. Air conditioner x Watt x Use Hours/Day
= 1 x 2000 x 7 = 14,000 Watt-Hour/Day
Power Consumption for Television = No. of Television x Watt x Use Hours/Day
= 1 x 110 x 5 = 550 Watt-Hour/Day
Power Consumption for Refrigerator = No. Refrigerator x Watt x Use Hours/Day
= 1 x 170 x 18 = 3,060 Watt-Hour/Day
Power Consumption for Freezer = No. Freezer x Watt x Use Hours/Day
= 1 x 275 x 18 = 4,950 Watt-Hour/Day
Power Consumption for Laptops = No. of Laptops x Watt x Use Hours/Day
= 2 x 95 x 4 = 760 Watt-Hour/Day
Power Consumption for Electric fan = No. Electric fan x Watt x Use Hours/Day
= 5 x 75 x 10 = 3,750 Watt-Hour/Day
Power Consumption for Sound system = No. Sound system x Watt x Use Hours/Day
= 1 x 200 x 1 = 200 Watt-Hour/Day
Power Consumption for Light bulbs = No. of Light bulbs x Watt x Use Hours/Day
= 20 x 10 x 8 = 1,600 Watt-Hour/Day
Power Consumption for Washing machine = No. Washing machine x Watt x Use Hours/Day
= 1 x 2400 x 1 = 2,400 Watt-Hour/Day
Total Electrical Load per Day
= 14,000 + 550 + 3,060 + 4,950 + 760 + 3,750 + 200 + 1,600 + 2,400
= 31,270 Watt-Hour/Day
Step-2: Calculate Electrical Power of the Loads (Wattage of Loads)
Most appliances have a wattage stamp located on the nameplate, bottom, or back of the device. The maximum power that the appliance can draw is indicated by its wattage. Many appliances have a range of settings, so the actual amount of power an appliance may consume depends on the setting being used.
Load for Air conditioner = No. Air conditioner x Watt
= 1 x 2000 = 2,000 Watt
Load for Television = No. of Television x Watt
= 1 x 110 = 110 Watt
Load for Refrigerator = No. Refrigerator x Watt
= 1 x 170 = 170 Watt
Load for Freezer = No. Freezer x Watt
= 1 x 275 = 275 Watt
Load for Laptops = No. of Laptops x Watt
= 2 x 95 = 190 Watt
Load for Electric fan = No. Electric fan x Watt
= 5 x 75 = 375 Watt
Load for Sound system = No. Sound system x Watt
= 1 x 200 = 200 Watt
Load for Light bulbs = No. of Light bulbs
= 20 x 10 = 200 Watt
Load for Washing machine = No. Washing machine x Watt
= 1 x 2400 = 2,400 Watt
Total Electrical Power of the Loads
= 2,000 + 110 + 3,060 + 4,950 + 760 + 3,750 + 200 + 1,600 + 1,600
= 5,920 Watt

Step-3: Calculate Size of Inverter:
With the total load calculated, we recommend an inverter size above the total wattage of the devices that will be running on the inverter, so there will be a safety margin and no risk of overloading.
Inverter Size = (Total Wattage x Safety Factor) / Efficiency
Total Electrical Load in Watt = 5,920 Watt
Total Electrical Load in VA = Watt / P.F
= 5,920 / 0.8 = 7,400VA
Size of Inverter = Total Load x Correction Factor / Efficiency
= 5,920 x 1.25 / 80% = 9,250 VA
≈10kVA
Step-4: Required Size of Batteries
(For Lead-acid batteries or Lithium-ion batteries)
Safety factor = 1.25
DoD for Lead-acid batteries = 50%
DoD for Lithium-ion batteries = 80%
Total Electrical Load per Day = 31,270 Watt-Hour/Day
For Lead-acid batteries
Usable Watt-Hour/Day of the Lead-acid battery bank
= Total Electrical Load per Day / DoD (% Efficiency)
= 31,270 x (100% / 50%) = 62,540Wh
For peak draw
= Usable Watt-Hour/Day of the Lead-acid battery bank x Safety factor
= 62,540Wh x 1.25 = 78,175Wh
Number of 12V 220AH Lead-acid batteries needed
= 78,175Wh / (12V x 220Ah) = 29.6 batteries
(for 48V set-up it must be multiple of 4 i.e. 28 or 32 batteries)
Best option is 32 batteries but cost more, that is extra 4 batteries.
To cutdown the cost…
≈ 28 pieces of Lead-acid batteries will be needed
For Lithium-ion batteries
Usable Watt-Hour/Day
= 31,270 / 0.8 = 39,087.5Wh
For peak draw
= 39,087.5 WH x 1.25 = 48,859.4Wh
We can use 5kWh, 10kWh, 7.5kWh or 15kWh 48V Lithium-ion batteries
Then,
48,859.4Wh / 5kWh = 9.8 ≈ 10 pieces
48,859.4Wh / 7.5kWh = 6.5 ≈ 6 or 7 pieces
48,859.4Wh / 10kWh = 4.9 ≈ 5 pieces
48,859.4Wh / 15kWh = 3.3 ≈ 3 pieces
Best option is to 5kWh or 10kWh Lithium-ion batteries but because of cost we will use 15kWh Lithium-ion battery
≈ 3 pieces of 15kWh Lithium-ion batteries
Step-5: Charging Current for Batteries
Now the Required Charging Current for these two types of batteries.
(Charging current should be 10% to 20% of batteries Ah)
For Lead-acid batteries
Battery bank power = 78,175Wh
Battery bank voltage = 48V
Then,
Ah = 78,175Wh / 48V
= 1,628.6Ah
Charging current
= Ah x 20%
= 1,628.6Ah x 20%
= 325.7A
Charging Time required for Batteries
Charging Time of battery = Battery Ah / Charging Current
T = Ah / A
= 1,628.6Ah / 325.7A = 5 Hours (Ideal Case)
It has been noted that 40% of losses occurred during the battery charging
The charging time required for 78,175Wh batteries, real case:
1,628.6Ah + 40%
Then, 1,628.6Ah x (40/100) = 651.4Ah
The battery rating would be 1,628.6Ah + 651.4 Ah
= 2,280 Ah (1,628.6Ah + losses)
Now the required charging time for the battery would be:
2,280Ah / 325.7A
= 7 Hours.
For Lithium-ion batteries
Battery bank power = 48,859.4Wh
System voltage = 48V
Then,
Ah = 48,859.4Wh / 48V
= 1,017.9Ah
Charging current
= 1,017.9Ah x 20%
= 203.6A
Charging Time required for Batteries
Charging Time of battery = Battery Ah / Charging Current
T = Ah / A
= 1,017.9Ah / 203.6A = 5 Hours (Ideal Case)
It has been noted that 40% of losses occurred during the battery charging
The charging time required for 48,859.4Wh batteries, real case:
1,017.9Ah + 40%
Then, 1,017.9Ah x (40/100) = 407.2Ah
The battery rating would be 1,017.9Ah + 407.2 Ah
= 1,425.1 Ah (1,017.9Ah + losses)
Now the required charging time for the battery would be:
1,425.1 Ah / 203.6A
= 7 Hours.
As you can see, the charging time for both Lead-acid and Lithium-ion batteries are the same. In the next step we shall see if both battery types will require the same wattage (number) of solar panels.
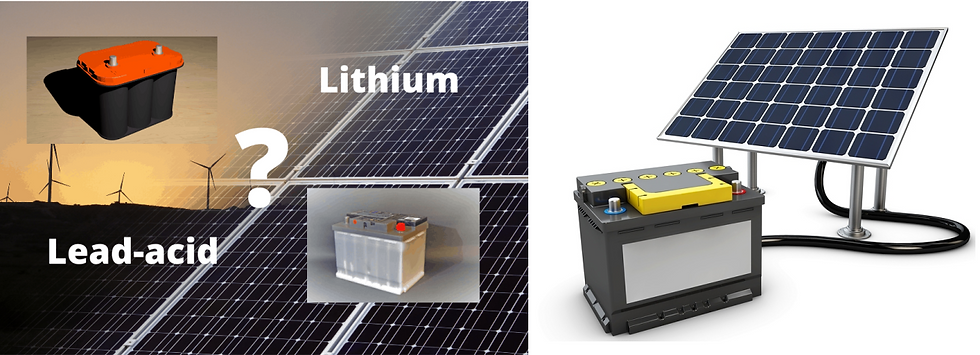
Step-6: Calculating The Solar Panel Size

Average Peak Sunshine Hours = 6 Hours
Total Electrical Load/Day = 31,270 Watt-Hour/Day
Safety Factor = 1.25
Required Size of Solar Panel
= (Electrical Load / Avg. Peak Sunshine) X Safety Factor
= (31,270Wh / 6h) x 1.25 = 6,514.6 Watt
Required Size of Solar Panel without battery bank
= 6,514.6 Watt
With Lead-acid battery bank
Battery bank power
= 78,175Wh
Total daily energy needed
= Total Electrical Load/Day + Battery bank power
= 31,270 + 78,175
= 109,445Wh
Required Size of Solar Panel
= 109,445Wh / 6h
= 18,240.8W
I.e., we need 18,240.8 W solar panels for the above explained system (This is for both Direct Load and Batteries Charging)
Now,
If we use 550W solar panels
18,240.8W / 550W = 33.2
≈ 33 pieces of 550W solar panels
With Lithium-ion battery bank
Battery bank power
= 48,859.4Wh
Total daily energy needed
= Total Electrical Load/Day + Battery bank power
= 31,270 + 48,859.4Wh
= 80,129.4Wh
Required Size of Solar Panel
= 80,129.4Wh / 6h
= 13,354.9W
I.e., we need 13,354.9W solar panels for the above explained system (This is for both Direct Load and Batteries Charging)
Now, if we use 550W solar panels
13,354.9W / 550W = 24.3
≈ 24 pieces of 550W solar panels
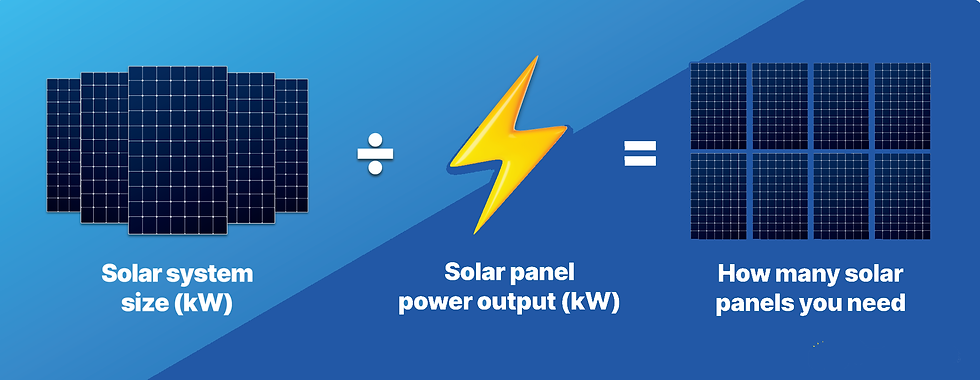
With Lead-acid battery bank
≈ 33 pieces of 550W solar panels are needed
With Lithium-ion battery bank
≈ 24 pieces of 550W solar panels are needed
Lithium-ion batteries offer a significant advantage in terms of solar power efficiency. They require less solar power to achieve the same stored capacity compared to lead-acid batteries. This translates to needing a slightly smaller solar panel system or getting more usable power out of your existing setup for charging lithium batteries.
Step-7: Calculating Solar PV String Size
A string is a series connection of multiple solar panels. While an array is a parallel connection of multiple string.
To calculating maximum string size
The maximum number of solar panels that can be connect in a string is determined by the maximum input voltage (MPP or Voc) of the inverter or charge controller divide by Open circuit voltage (Voc) of solar panel. You can find MPP value on the inverter or charge controller datasheet.
Maximum input voltage (MPP) of charge controller
MPP = 195V
For our 550W solar panels
Open circuit voltage: Voc = 49.80V
Derating factor = 0.80
Maximum string size
= (MPP / Voc) x 0.80
= 195 x 0.80 / 49.80
= 3.1
≈ 3 panels per strings
So, this means if you connected 3.1 panels to the MPPT you would be right at the charge controller’s voltage limit. Now obviously we can’t have 0.1 of a panel, so we always round down to the nearest whole number. In this case, 3 panels per string is the maximum.
Also, 3 panels string voltage will not exceed maximum limit of the MPPT
Check: 3 x 49.80V = 149.4V < MPP (195V)
But 4 x 49.80V = 199.2V > MPP
Solar PV String Size
= 3 panels per strings
For Lead-acid battery bank
String connection = 3 panels in series
Array connection = 11 x 3 panels in series <> (33 panels / 3 = 11 panels)
Then,
Panels configuration = 3S 11P
Lithium-ion battery bank
String connection = 3 panels in series
Array connection = 8 x 3 panels in series <> (24 panels / 3 = 8 panels)
Then,
Panels configuration = 3S 8P
Calculating minimum string size
The method is very similar to calculating maximum string size. The main difference is that Vmp is used instead of Voc of the solar panel
For our 550W solar panel
· Vmp = Voltage at max Power (Vmp) = 41.95V
Now we will use minimum end of the MPP (minimum input voltage of MPPT or Inverter) range. MPP = 60V
Derating factor = 0.80
Minimum string size
= (MPP / Vmp) x 0.80
= 60 x 0.80 / 41.95 = 1.14
≈ 2 panels per stings
In this case, a fraction of panel cannot be connected, so we round up to 2 panels.
Check: A single panel 41.95V < 60V (MMP)
But 2 x 41.95V = 83.9V > 60V
The aim is to find the voltage that will fall within the minimum and maximum input of the MPPT
Based on these calculations, we have a string size of minimum of 2 panel and a maximum of 3 panels.
Step-8: Calculating the rating of charge controller:
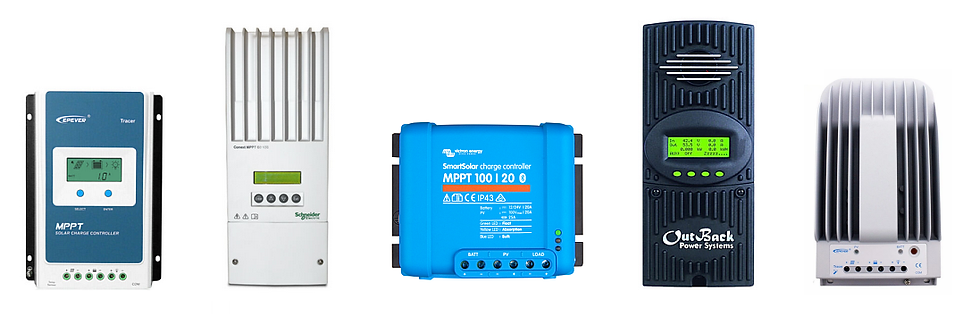
This is a simple equation.
Watts of panels / Battery bank Volts = Amps of charge controller.
The total watts of the solar array divided by the voltage of the battery bank. That will give the output current of the charge controller.
For Lead-acid batteries
Solar panels array power = 18,240.8W
Battery bank voltage = 48V
Total Amps of MPPT
= 18,240.8W / 48V
= 380A
If we choose to use 100A MPPT charge controllers
Then,
380A / 100A = 3.8 ≈ 4
Check: 380A / 4 = 95A (<100A)
That’s 4 x 100A MPPT will be needed to charge the 28 x 12V x 220AH Lead-acid batteries
Practically
Configuration of solar panels (if a single MPPT is used)
= 3S 11P
To connect 33 solar panels to the 4 MPPTs
33 / 4 = 8.25 solar panels
Since we cannot connect 0.25 panel and the string connection is 3 panels, there is no way to connect strings of 3 panels with 8.25 panels.
For proper configuration, we will around up the solar panels to 9 solar panels for each of the MPPTs
Then,
New configuration will be 3S 3P for each of the 4 MPPTs
For our 550W Solar Panel, the
Short Circuit Current (Isc/A) = 13.98A
Total current in paralleling 3 strings is Isc x 3
Then,
Isc x 3 = 13.98 x 3 = 41.94A
Therefore, an MPPT with 45A looks like it will handle the current, but its maximum input voltage must be considered.
For 45A, 60A and 80A MPPT available to us, the max input voltage is 145V
For a strings of 3 panels
Voltage = Voc x 3 = 49.80V x 3 = 149.4V > 145V of the MPPT (max input)
For safety, an MPPTs with 150V and above are recommended. Also, for future expansion 100A/120A MPPT is recommended.
Check, max power of 3S 3P
= 3 x 3 x 550W = 4,950W
System voltage
= 48V
Derating factor
= 0.80
Then,
Current = 4,950 x 0.80 / 48V
= 82.5A (confirmed: MPPT of 100A upward is recommended)
We shall see if the lithium-ion batteries will accept MPPTs less than 100A
For Lithium-ion batteries
Solar panels array power = 13,354.9W
Battery bank voltage = 48V
Total MPPT Amps = 13,354.9W / 48V
= 278.2A
If we choose to use 100A MPPT charge controller
Then,
278A / 100A = 2.8 ≈ 3
Check: 278A / 3 = 92.6A (<100A)
That’s 3 x 100A MPPT will be needed to charge 3 x 15KWH Lithium-ion batteries
Practically
Configuration of solar panels (if a single MPPT is used)
= 3S 8S
To connect 24 solar panels to the 3 MPPTs
24 / 3 = 8 solar panels
Then,
New solar panels connection to the 3 MPPTs
For each set of 8 panels, 2S 4P or 4S 2P can be connected to each of the 3 MPPTs but maximum input voltage must take into consideration, so 4S 2P will not work.
Check: voltage of 4S 2P
= 4 x Voc of solar panel
= 4 x 49.80 = 199.2 > max input of our MPPT (195V)
So, the configuration will be 2S 4P for each of the 3 MPPTs
Total current in paralleling 2 strings is
= Isc x 4
For our 550W Solar Panel, the Short Circuit Current (Isc/A) = 13.98A
Isc x 4 = 13.98 x 4 = 55.92A
Therefore, an MPPT with 60A looks like it will handle the current, but its maximum input voltage must be considered
For 60A and 80A MPPT available to us, the max input voltage is 145V
For a strings of 2 panels
Voltage = Voc x 2 = 49.80V x 2 = 99.6V < 145V of the MPPT (max input)
Also 99.6V > 60V of MPPT minimum input voltage.
Considering cost, MPPTs with 60A up may work but maximum input power of the MPPT must be looked into
Check, max power of 2S 4P
= 2 x 4 x 550W = 4,400W
System voltage
= 48V
Derating factor
= 0.80
Then,
Current = 4,400 x 0.80 / 48V
= 73.3A (confirmed: MPPT of 80A upward is recommended)
As you can see, 60A MPPT cannot handle power of 8 x 550W even though the voltage of the series and current of parallel arrangement seems to be within the range
Future Expansion: If you plan to add more solar panels in the future, choose (100A and 120A) an MPPT controller with sufficient current capacity to handle the potential increase in power output.
Step-9: Calculating the wire size for the set-up:
For both performance and safety concerns, selecting the appropriate DC wire diameters for the solar power system is crucial. The size of the cables must be appropriate for the voltages and currents the system uses.
Important note:
If the wires are undersized, there will be a significant voltage drop in the wires resulting in excess power loss.
In addition, if the wires are undersized, there is a risk that the wires may heat up to the point in which a fire may result.
The following requirements must be met by the wires:
1. The voltage rating must match or exceed the system's voltage rating
2. The current carrying capacity must match or exceed the current to be carried
3. The wires must be able to withstand environmental conditions
4. Special attention to be paid to voltage drop.
5. It’s very important to consider how long the wire needs to be
Calculating Wire Size for Solar Panels
(Between Solar Panel and Charge Controller)
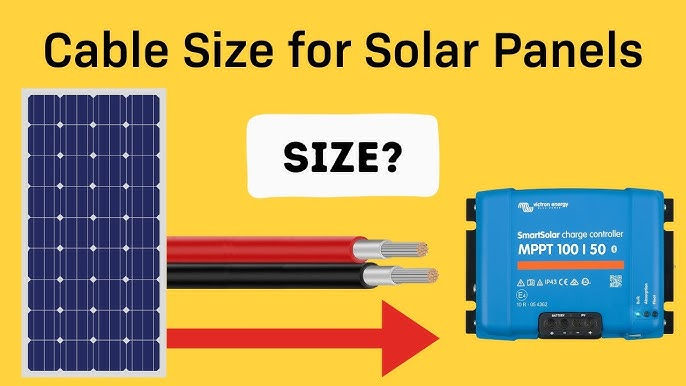
The current capacity required for the wire:
Wire Amp Rating ≥ Number of solar panels in parallel (Np) × Short Circuit Current (Isc) x Safety Factor
For our 550W Solar Panel
Short Circuit Current (Isc/A) = 13.98A
Safety Factor = 1.25
Then,
Imax (wire) ≥ Np x Isc x safety factor
For Lead-acid batteries setup
Each MPPT 3S 3P
Imax ≥ 3 x 13.98 x 1.25
= 52.42A
For Lithium-ion batteries
Each MPPT 2S 4P
Imax ≥ 4 x 13.98 x 1.25
= 69.9A
The maximum currents that can flow through the wires have been determined, if the distance between the solar panels and MPPT charge controllers (length of wires) is 25metres.
We can now use ampacity table or/and online calculator but with caution
Wire ampacity tables: These tables list the maximum safe current a specific wire size can carry under different temperature conditions. You can find them online or in electrical reference materials.
Online calculators: Some online calculators can help with voltage drop calculations and recommend wire sizes based on your input parameters. However, use these tools with caution and consult a qualified electrician for verification.
For 48V setup, current of 52.42A to 69.9A and a distance of 20m a wire size of 25mm² is recommended to ensure minimal voltage drop and safe operation.
If the distance is above 25m ≤ 30m please consider wire size of 35mm²
To sum up, choosing the right type and size of cable for your off-grid solar system is essential for effective energy transmission and reducing resistive losses. The minimal wire size needed for a given distance can be found using the wire Amp rating, voltage drop formula and wire gauge table by taking into account variables such the maximum current, safety factor, and voltage drop.
The lifetime and functionality of your off-grid solar system can also be increased by making an investment in premium cables and using the right installation methods. Better wires can resist more severe weather and lower the chance of electrical fires and system malfunctions.
Calculating Wire Size
Between Charge Controller and Battery Bank
To calculate the current capacity required for the wire:
Wire Amp Rating ≥ Charge Controller Rated Amps x 1.25
We will take the upper ratting 69.9A (calculated before: Imax of wire between PV and MPPT)
Then,
Wire Amp Rating ≥ 69.9A x 1.25
≥ 87.38A
Between Battery Bank and Inverter
To calculate the current capacity required for the wire:
Wire Amp Rating ≥ Inverter Continuous Power Rating / (% Peak Efficiency x System Voltage) x Safety Factor
Inverter power = 5,920 W
% Peak Efficiency = 80%
System Voltage = 48V
Safety Factor = 1.25
Wire Amp Ratin ≥ 5,920W / (0.8 x 48V) x 1.25
≥ 192.7A
In between Batteries
Determine the maximum continuous charge/discharge current of the battery first. Refer to the battery's specifications for this information.
This information can be found on the battery's datasheet
To calculate the current capacity required for the wire between batteries:
Wire Amp Rating ≥ Battery Max Continuous Charge/Discharge Current x Safety Factor (1.25)
Step-10: Calculating DC Circuit Breakers Ratings:
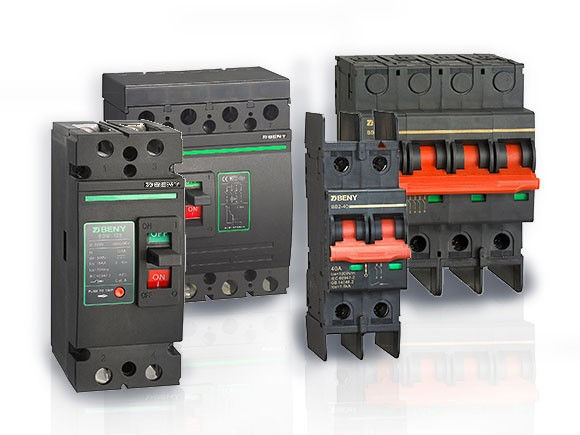
Circuit breakers and fuses are used to prevent overheating of the wire and to prevent any connected device in the system from burning or being harmed in the event of a short circuit. It is designed to guard against damage from short circuits or overvoltage in the system. Although fuses or circuit breakers are not required for the system to function correctly, we always advise using them for safety.
Circuit breakers facilitate routine maintenance and repairs. They allow solar technicians to work on specific parts of the system without needing to shut down the entire array, enhancing safety and minimizing downtime.
We advise placing fuses or circuit breakers in three distinct locations. Number 1 and 2 of those locations are absolutely necessary.
1. Between solar panels and a charge controller
2. Between a battery bank and an inverter
3. Between a charge controller and a battery bank
Between Solar Panels and A Charge Controller
In our case, we have more than one MPPT
Circuit breaker between solar panels and a charge controller will be sized based on the maximum current flowing through the circuit breaker.
The maximum currents for solar panels should be of 1.25 (safety factor) times the short circuit currents of the solar panels.
For Lead-acid battery bank
We have 4 MPPTs
And the panel configuration is 3S 3P for each of the 4 MPPTs
Total current for each array
= Isc x 3
For our 550W Solar Panel, the Short Circuit Current (Isc/A) = 13.98A
Isc x 3 = 13.98 x 3 = 41.94A
Then,
Circuit Breaker Ratting = 41.94A x 1.25 = 52.43A
The closest standard breaker size is 60A (63A)
Therefore, we need 4 of 60A circuit breakers between each MPPT and its solar array
For Lithium-ion battery bank
We have 3 MPPTs
And the panel configuration is 2S 4P for each of the 3 MPPTs
Total current for each array
= Isc x 4
For our 550W Solar Panel, the Short Circuit Current (Isc/A) = 13.98A
Isc x 4 = 13.98 x 4 = 55.92A
Then,
Circuit Breaker Ratting = 55.92A x 1.25 = 69.9A
The closest standard breaker size is 80A
Therefore, we need 3 of 80A circuit breakers between each MPPT and its solar array
Between A Battery and An Inverter
Circuit Breaker Rating = Inverter Watts / (Battery Rated Voltage x Peak Inverter Conversion Efficiency) x 1.25
Inverter Watts = 5,920 Watt
Battery Voltage = 48 Volt
Efficiency = 0.8
Safety Factor = 1.25
Circuit Breaker Rating = 5,920 / (48 x 0.8) x 1.25 = 192.71A
The closest standard breaker size is 200A
Between A Charge Controller and A Battery
Circuit Breaker Rating = Rated Charge Current of the Charge Controller x 1.25
MPPT Amp = 100A
Safety Factor = 1.25
Circuit Breaker Rating = 100A x 1.25 = 125A
The unsung heroes of solar systems are circuit breakers, which guarantee effective functioning and offer a crucial layer of defense against electrical risks.
They are an essential part of every solar installation because of their capacity to recognize and react to anomalous conditions.
A safer and more sustainable future requires using high-quality circuit breakers, not merely as a choice as more people adopt solar power as a greener energy source.
Step-11: Calculating DC Surge Protection Device for the Solar Power:
Solar arrays without surge protection devices (SPDs) are more susceptible to damage from surges current and transient spikes voltage.
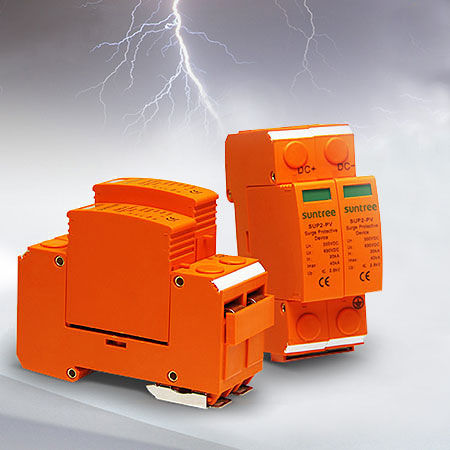
It is important to protect both AC & DC sides from lightning strikes by using a proper solar surge protection device.
There are two versions of SPD: one for households, which works on AC, and one for solar, which we are discussing here. Ensure you get the DC version for your solar input.
Consider also, whether you need an AC or DC SPD. Each has its place in solar systems and must be used accordingly. In essence, an AC SPD will be used for alternating current (AC) circuits of the solar system, while DC SPDs are used for direct current (DC) circuits.
Types Of Solar Surge Protection Device (SPD)
A solar system will usually be installed with several surge protection devices, right from the solar panels to the load. The three most common surge protection device types are listed below:
Type 1 SPD: These devices are designed to protect against surges that come from outside of the system such as solar arrays.
Type 2 SPD: These protect against surges that come caused by indirect lightning strikes. (Reduces overvoltage(s) coming from numerous sources.
Type 3 SPD: Type 3 protection devices are meant to protect specific types of equipment from surges. They are usually installed in the vicinity of the equipment or load.
To choose a proper SPD model for the solar/PV system, the following points should be kept in mind:
Lightning round flash density.
System’s operating temperature.
System’s voltage.
System’s short circuit current rating.
Level of the waveform that is to be protected against
Nominal discharge current.
Voltage Limits:
DC SPDs are designed to limit surge voltage levels to safe thresholds. This voltage limit ensures that overvoltage(s) do not exceed the load capacity of the device. By keeping the voltage within a safe range, the DC SPD protects the system from damage and helps prevent equipment failure.
The maximum continuous operating voltage Uc PV of the photovoltaic system: usually DC 500V 600V 800V 1000V 1200V 1500V
To determine the maximum nominal voltage required for the photovoltaic system. It should match or exceed the maximum voltage that the panel will produce. Residential systems are typically rated at 1000 V or less, but commercial or public systems may require a higher voltage rating. Hence a DC SPD 1000V can be applied.
Selecting operating voltage (Uc) for DC Surge Protection Device for Solar Panel:
Uc = (Peak Voltage x 2) + 10%
If we take Peak Voltage = Maximum input voltage (MPP) of MPPT
Then,
Uc = (195V x 2) + 10% = 390V + 39V = 429V
We can comfortably use DC SPD with Uc = 500V
Match the SPD to the inputs of your power conversion equipment you want to protect by ensuring the “Uc” voltage in the SPD datasheet is at or just slightly above the maximum continuous voltage on the conductors to be protected, or the maximum voltage rating of the power equipment connected. If the SPD’s “Uc” rating is well above the maximum voltage rating of the power equipment connected, it can no longer effectively protect from voltage surges. The SPD will protect devices or equipment by activating well above the maximum continuous operating voltage “Uc” and will not interfere at voltages below “Uc”.
Voltage Protection Level (Up):
Up is a measure of how effectively a DC SPD limits transient voltages. This represents the voltage level at which the solar surge protection device limits transient voltages. Choose a DC SPD with a lower VPL as it provides better protection for your system.
Installation of DC SPD
At Main Service Entrance:
Install a Type 1 DC SPD at the service entrance of the building to protect the entire electrical system, including the solar array. Connect the DC SPD in parallel with the input power supply. Follow the manufacturer’s instructions for proper wiring and connections.
Installing a Sub-district DC SPD:
Especially in large photovoltaic systems, Type 2 DC SPDs are installed in sub-distribution boards or collector boxes. These DC SPDs provide local protection for circuits connected to the panel. Connect the DC SPD in parallel in the circuit to ensure proper wiring and alignment with the main service input DC SPD.
Device-level DC SPD Installation:
Depending on system requirements and device sensitivity, you should consider installing Type 3 DC SPDs in specific device layers such as inverters and charge controllers. These DC SPDs provide an additional layer of protection at the device level. Follow the manufacturer’s instructions for proper installation and connection.
The purpose of a surge protective device is to shunt and suppress transient voltages being introduced into an electrical distribution system from either an external or internal source. Selecting the proper surge current-rated SPD throughout the electrical distribution system provides the best performance life for equipment.
Conclusion:
Off-grid solar power offers a chance to harness clean energy and potentially cut your electricity costs. While the upfront investment might seem high, the long-term savings can outweigh the initial cost.
Yes, off-grid solar has drawbacks like limited scalability and upfront costs. But the benefits including energy independence, environmental friendliness, and reliability are significant. To decide if it's right for you, consider your energy needs, location, and budget. With careful planning and professional help, off-grid solar can be a sustainable path to a greener future.
By carefully weighing the benefits and drawbacks, and with proper planning and implementation, off-grid solar systems can be a viable and sustainable solution for a brighter, greener future. They empower you to take control of your energy consumption and contribute to a more sustainable world, all while potentially saving money in the long run.
Comments